CRISPR-Cas9: a powerful and precise genomic editing tool.
Laboratory of Biotoxicology, Pharmacognosy and biological valorisation of plants, Faculty of Sciences, Department of Biology, University of Saida - Dr Tahar Moulay, 20100 Saida, Algeria.
Abstract
CRISPR-Cas9 is a gene editing technology that uses a small RNA molecule and a protein called Cas9 to make precise changes to the genome of living organisms. It has numerous potential applications, including the treatment of genetic diseases, the production of crops with improved traits, and the creation of new drugs and therapies. However, the technology has also raised ethical concerns about potential misuse and long-term effects on health. Scientists and policymakers are working to establish guidelines and regulations for its responsible and ethical use. In addition to its medical and agricultural applications, CRISPR is also being used for basic scientific research to gain insights into the function of specific genes and their role in development and disease.
Key words
CRISPR, Cas, genome editing, editing technology, CRISPR applications.
Introduction
CRISPR1️⃣ -Cas92️⃣ , often shortened as just CRISPR, is a revolutionary technology that has transformed the field of genetics and molecular biology. It is a tool that allows scientists to precisely and efficiently edit the genome of living organisms, including plants, animals, and humans (Hwang et al., 2017; Liang et al., 2015; Mali et al., 2013). The ability to manipulate the genetic code of living things has opened up numerous possibilities for research and applications, including the potential to develop new treatments for diseases and to improve crop yields. Since its discovery in 2012 (Jinek et al., 2012), CRISPR has been widely studied and has already had a significant impact on the scientific community. It has the potential to continue to revolutionize the way we understand and interact with the natural world.
The CRISPR genome editing system has been adopted from a naturally occurring bacterial defense system (immunity system).
During viral infection, bacteria extract small genome pieces from the invading viruses' DNA and insert
them into their own DNA in specific arrangement creating segments known as CRISPR archives or arrays.
The bacteria then use the CRISPR arrays to recognise the viruses or those closely related ones in the
event of recurring viral invasion. In such cases, bacteria translate the relevant CRISPR segments into
short RNA sequences, guide RNA (gRNA) that can help target and bind to specific regions of the viruses' DNA genome.
The bacteria then halt the viral attack by cutting the target DNA region using the Cas9 or similar enzymes.
So, what is CRISPR and what can it be used for, questions that we will try to answer in a few lines.
1️⃣ CRISPR: Clustered Regularly Interspaced Short Palindromic Repeats.
2️⃣ Cas9: CRISPR associated protein 9 (one of the proteins necessary for the CRISPR system to function).
General overview of CRISPR and its applications
CRISPR works by using a small RNA molecule to guide a protein called Cas9 to a specific location in the genome. Once there, the Cas9 protein can make a cut in the DNA, which allows scientists to insert, delete, or modify specific sequences of DNA (
Fig.1). This process is called CRISPR-Cas9 genome editing (Doudna & Charpentier, 2014; Jinek
et al., 2012; Liu
et al., 2014). One of the main benefits of CRISPR is its accuracy and efficiency. It allows scientists to make precise changes to the genome with a high degree of control and can be used to target specific genes or regions of the genome. This has opened up a whole new field of research and has numerous potential applications, including the treatment of genetic diseases, the production of crops with improved traits, and the creation of new drugs and therapies (Doudna & Charpentier, 2014; Mali
et al., 2013). One of the most well-known applications of CRISPR is the treatment of genetic diseases. Many genetic diseases, such as sickle cell anemia and cystic fibrosis, are caused by mutations in a single gene (Platt
et al., 2014). By using CRISPR to edit the genome, scientists can potentially correct these mutations and restore the normal function of the gene (Mao
et al., 2017; Porteus & Takahashi, 2015). This has the potential to significantly improve the lives of individuals suffering from these diseases (Baliou
et al., 2018).
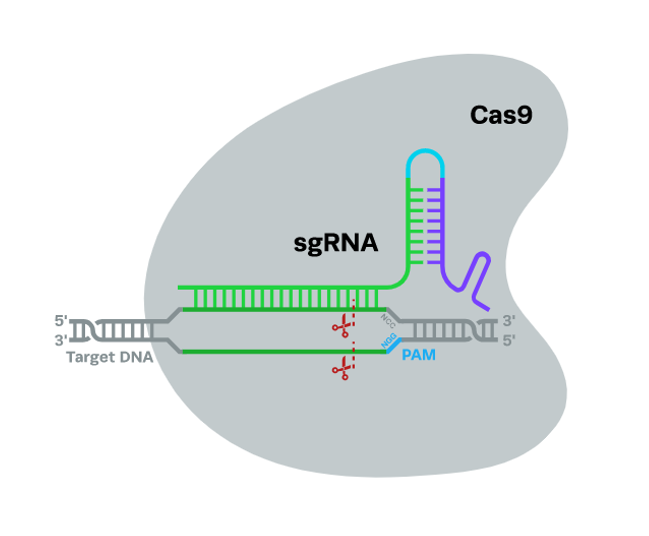
Fig.1. The CRISPR-Cas9 system.
There are sequences in the bacterial genome known as clustered regularly interspaced palindromic repeats (CRISPR). When coupled with a number of CRISPR-associated proteins, they provide defense against invasive viruses. One of the related proteins, Cas9, is an endonuclease that cleaves DNA along both strands. An RNA segment directs Cas9 to its target. Synthetic single guide RNA (sgRNA) is a single strand that can be produced; the portion of the RNA that binds to the genomic DNA is between 18 and 20 nucleotides long. The protospacer adjacent motif is a specific sequence of 2–5 nucleotides (PAM) that must be present at the 3' end of the guide RNA in order for Cas9 to cut the DNA. The precise sequence varies on the bacterium that generates Cas9.
CRISPR is also being used in the field of agriculture to produce crops with improved traits, such as increased resistance to pests and diseases. This can help to reduce the use of pesticides and herbicides, which can be harmful to the environment and human health (Klimentidis et al., 2017). In addition, CRISPR is explored as a tool for creating new drugs and therapies. For example, according to Doudna and Charpentier (2014), scientists are using CRISPR to modify the genomes of microorganisms to produce valuable compounds, such as enzymes and antibiotics. This can potentially lead to the development of new and more effective drugs. In a study by Chen et al. (2013), CRISPR-Cas was demonstrated to have the potential to be used for dynamic imaging of genomic loci in living human cells, which has implications for drug development and therapy. A review by Gaj et al. (2013) also discusses the potential of CRISPR-Cas as a genome engineering tool for drug development and therapy.
However, despite the many potential benefits of CRISPR,
the system suffers from a number of limitations the cheif of is the off-target effects which are of major concern in
eukaryotic organisms for therapeutic applications (Yang et al., 2018, Zischewski et al., 2017). The targeting specificity of CRISPR depends on the gRNA of Cas9
and what are known as PAM sequences (Protospacer Adjacent Motif sequences), and off-target cleavage in the genome (Suleiman et al., 2020).
The technology has also raised ethical concerns. One concern is the potential for misuse, such as the use of CRISPR to create designer babies or to enhance human traits (Hsu, Lander, & Zhang, 2014). There are also concerns about the potential long-term effects of genome editing, as it is not yet fully understood how these changes may affect the health and development of individuals in the long term (Hsu, Lander, & Zhang, 2014).
To address these concerns, scientists and policymakers are working to establish guidelines and regulations for the use of CRISPR. These efforts aim to ensure that the technology is used responsibly and ethically, while still allowing for its potential benefits to be realized (Scheufele et al., 2017). In addition to its potential uses in medicine and agriculture, CRISPR is also being explored as a tool for basic scientific research. By using CRISPR to manipulate the genome of model organisms such as mice and fruit flies, scientists can gain insights into the function of specific genes and their role in development and disease. This has the potential to greatly advance our understanding of genetics and molecular biology (Gaj et al., 2013).
Conclusion
Overall, CRISPR is a powerful and transformative technology that has the potential to revolutionize the fields of genetics and molecular biology. It has already had a major impact and will continue to shape the future of medicine and agriculture in the years to come. The development of CRISPR has also sparked a wave of innovation in the field of genome editing, with the development of new CRISPR-based technologies such as CRISPR-Cpf1 and CRISPR-Cas12a (Paul et al., 2020; Yang et al., 2020). These technologies have the potential to expand the capabilities of CRISPR and further broaden its applications in research and medicine.
Useful link
A more explanatory video on how the CRISPR-Cas9 system works can be found at the link:
References
Baliou, S., Adamaki, M., Kyriakopoulos, A. M., Spandidos, D. A., Panayiotidis, M., Christodoulou, I., & Zoumpourlis, V. (2018). CRISPR therapeutic tools for complex genetic disorders and cancer. International journal of oncology, 53(2), 443-468.
Chen, B., Gilbert, L. A., Cimini, B. A., Schnitzbauer, J., Zhang, W., Li, G. W., & Liu, D. R. (2013). Dynamic imaging of genomic loci in living human cells by an optimized CRISPR/Cas system. Cell, 155(7), 1479-1491.
Chen, J. S., Gilbert, L. A., Cimini, B. A., Schnitzbauer, J., Zhang, W., Li, G. W., & Liu, D. R. (2013). Dynamic imaging of genomic loci in living human cells by an optimized CRISPR/Cas system. Cell, 155(7), 1479-1491.
Doudna, J. A., & Charpentier, E. (2014). The new frontier of genome engineering with CRISPR-Cas9. Science, 346(6213), 1258096.
Gaj, T., Gersbach, C. A., & Barbas, C. F. (2013). ZFN, TALEN, and CRISPR/Cas-based methods for genome engineering. Trends in Biochemical Sciences, 38(7), 391-400.
Hsu, P. D., Lander, E. S., & Zhang, F. (2014). Development and applications of CRISPR-Cas9 for genome engineering. Cell, 157(6), 1262-1278.
Hwang, W. Y., Fu, Y., Reyon, D., Maeder, M. L., Tsai, S. Q., Sander, J. D., & Joung, J. K. (2017). Efficient genome editing in human pluripotent stem cells using Cas9 from Neisseria meningitidis. Nature Protocols, 12(8), 1971-1982.
Jinek, M., Chylinski, K., Fonfara, I., Hauer, M., Doudna, J. A., & Charpentier, E. (2012). A programmable dual-RNA-guided DNA endonuclease in adaptive bacterial immunity. Science, 337(6096), 816-821.
Klimentidis, Y. C., Beyer, A. D., & Saxton, A. M. (2017). The potential of CRISPR-Cas9-based gene editing to revolutionize agriculture. Frontiers in genetics, 8, 44.
Liang, P., Xu, Y., Zhang, X., Ding, C., Huang, R., Zhang, Z., & Liu, J. (2015). CRISPR-Cas9-mediated gene editing in human tripronuclear zygotes. Protein & Cell, 6(5), 363-372.
Liu, H., Kim, J. Y., & Kim, J. S. (2014). Targeted genome engineering in human cells with the Cas9 RNA-guided endonuclease. Nature Protocols, 9(1), 23-36.
Mao, Y., Zhang, C., Xiong, S., Ni, Y., Chen, J., Wang, M., & Chen, Y. (2017). Correction of sickle cell disease-associated mutation in human hematopoietic stem/progenitor cells by CRISPR/Cas-9. Cell Stem Cell, 20(6), 811-817.
Mali, P., Yang, L., Esvelt, K. M., Aach, J., Guell, M., DiCarlo, J. E., & Church, G. M. (2013). RNA-guided human genome engineering via Cas9. Science, 339(6121), 823-826.
Paul, B., & Montoya, G. (2020). CRISPR-Cas12a: Functional overview and applications. biomedical journal, 43(1), 8-17.
Platt, R. J., Chen, S., Zhou, Y., Yim, M. J., Swiech, L., Kempton, H. R., & Liu, D. R. (2014). CRISPR-Cas9 knockin mice for genome editing and cancer modeling. Cell, 159(2), 440-455.
Porteus, M. H., & Takahashi, K. (2015). Gene therapy for inherited disorders: progress and challenges. Nature, 526(7575), 311-318.
Scheufele, D. A., Xenos, M. A., Howell, E. L., Rose, K. M., Brossard, D., & Hardy, B. W. (2017). US attitudes on human genome editing. Science, 357(6351), 553-554.
Suleiman AAJ, Saedi WY, Muhaidi MJ. Widely used gene editing strategies in cancer treatment a systematic review. Gene Rep. (2020) 1:100983–99.
Yang HC, Chen PJ. The potential and challenges of CRISPR-Cas in eradication of hepatitis B virus covalently closed circular DNA. Virus Res. (2018) 244:304–10.
Yang, Z., Edwards, H., & Xu, P. (2020). CRISPR-Cas12a/Cpf1-assisted precise, efficient and multiplexed genome-editing in Yarrowia lipolytica. Metabolic engineering communications, 10, e00112.
Zischewski J, Fischer R, Bortesi L. Detection of on-target and off-target mutations generated by CRISPR/Cas9 and other sequence-specific nucleases. Biotechnol Adv. (2017) 35:95–104.